Indium Phosphide Wafer (InP)
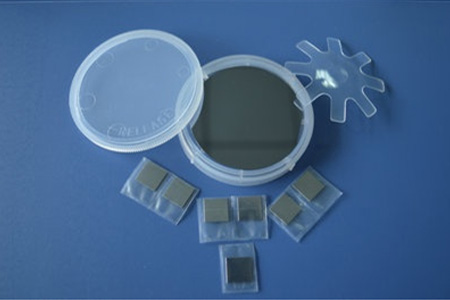
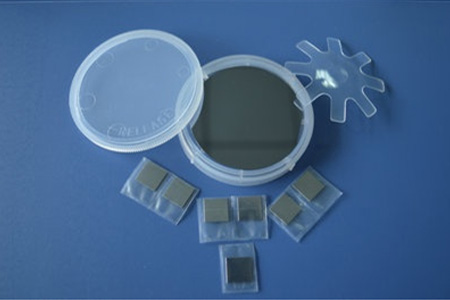
Indium Phosphide Wafer
Indium phosphide (InP) is an important compound semiconductor material with the advantages of high electronic limit drift speed, good radiation resistance, and good thermal conductivity. The materials are very suitable for manufacturing high frequency, high speed, high power microwave devices, and integrated circuits. It's widely used in solid-state lighting, microwave communication, fiber-optic communication, solar cells, guidance/navigation, satellite, and other civil and military fields.
Indium Phosphide Wafer Physical Properties
Material | InP |
---|---|
Growth Method | LEC,VCZ/P-LEC , VGF, VB |
Lattice (A) | a=5.869 |
Structure | M3 |
Melting Point | 1600℃ |
Density(g/cm3) | 4.79 g/cm3 |
Doped Material |
Undoped
S-doped
Zn-doped
Fe-doped
|
Type |
N
N
P
N
|
Carrier Concentration (cm-3) |
(0.4-2) x 1016
(0.8-3) x 1018
(4-6) x 1018
(0.6-2) x 1018
107-108
|
Mobility (cm2v-1s-1) |
(3.5-4) x 103
(2.2-2.4) x 103
(1.3-1.6) x 103
70-90
≥2000
|
EPD (Average) |
<5 x 104/cm2
3 x 104/cm2
2 x 103/cm2
2 x 104/cm2
3 x 104/cm2
|
Indium Phosphide Wafer Specifications
Size | 10 x 10 x 0.35mm, 10 x 5 x 0.35mm, 2'' Dia, 3'' Dia,4' Dia (customized sizes are available) |
---|---|
Thickness | 0.35 mm, 0.6 mm |
Polished | SSP or DSP |
Orientation | <100>, <111> |
Redirection Precision | ±0.5° |
Primary Flat Length | 16±2 mm, 22±2 mm, 32.5±2 mm |
Scondary Flat Length | 8±1 mm, 11±1 mm, 18±1 mm |
TTV | <10 um, <15 um |
Bow | <10 um, <15 um |
Warp | <15 um |
Indium Phosphide Wafer Package
Packaged with class 100 clean bag or wafer container in a class 1000 clean room.
Related Products of Indium Phosphide Wafer
Magnetic Ferroelectricity Substrates |
Semiconductor Wafers |
|
GaN Thin Film Substrates |
Halide Substrates |
Ceramic Substrates |
FREE QUOTE


